Wind Engineering
The race to master flight had pushed the development of fluid dynamics and its tools to the forefront in 20th century. Engineers and designers soon applied these concepts to improve other aspects of our lives. As we built taller and bigger structures to meet our needs, it became apparent that aerodynamics would play a role in their design as well. Tragedies such as the 1879 Tay Bridge Disaster in Scotland motivated engineers and designers to begin paying attention to how the wind interacted with their structures and how it influenced the surroundings. The field of wind engineering was thus born. CPP provides industry-leading wind consulting services to architects, engineers, and developers around the world. Such services are an integral part of the development process of the urban landscape, aiding us in our quest to build ever taller structures and also in more simple considerations, like ensuring our favourite outdoor cafe gets just the right breeze. Other interesting aerodynamics investigated at CPP include the aerodynamics of the interactions between the support gantries and launch vehicles for space missions.
Dr. Roy Denoon
For this blog, we are delighted to have interviewed Dr Roy Denoon, Vice President at CPP Wind Engineering. He leads CPP's structural wind loading team and is an industry specialist in wind engineering for tall buildings. Prior to his current post at CPP in Denver, Colorado, he spent his undergraduate years at the University of Edinburgh studying civil engineering, before obtaining an MEng(Res) and finally his PhD in civil engineering from the University of Sydney and University of Queensland, respectively. Dr Denoon has applied wind engineering principles on iconic buildings such as the Marina Bay Sands in Singapore and the International Commerce Centre in Hong Kong. Outside of his work, Roy enjoys cycling and has channelled his interest in aerodynamics to improve all aspects of cycling, having been involved in testing the aerodynamics of cycles, cycle components, testing methodologies and even designing cycle specific wind tunnels. In his own words, "I have used the knowledge of aerodynamics to field test and improve my position and I admit, my aerodynamics have yielded me better results than my talent!"
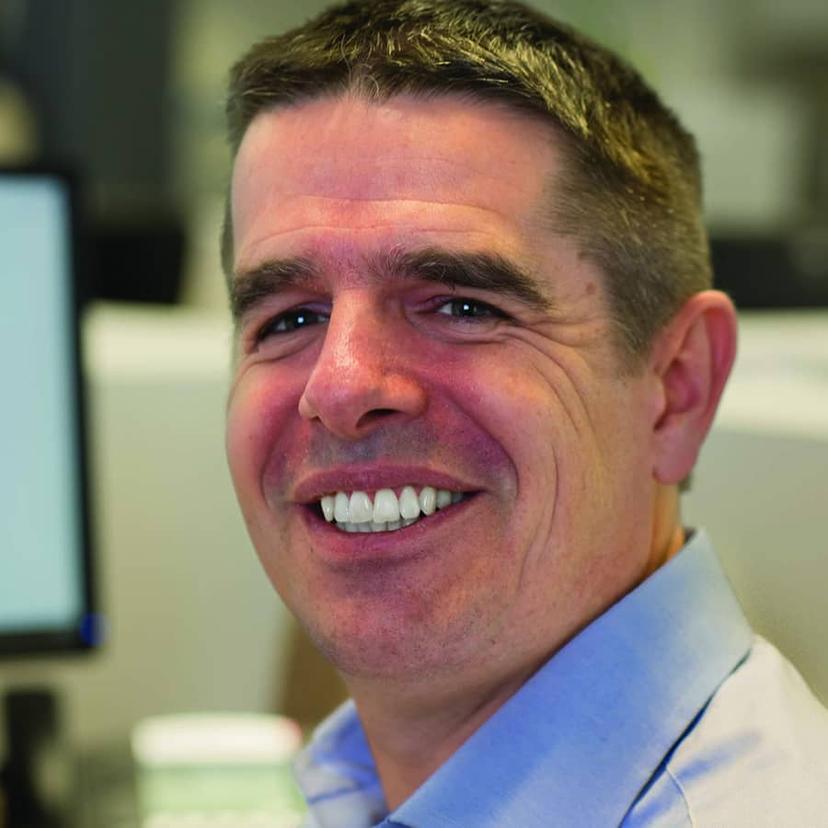
Aerodynamics of buildings
In the beginning
From the early days of wind engineering, incidents such as the 1879 Tay Rail Bridge disaster in Scotland highlighted the importance of wind loading on structures. An entire section of a rail bridge had collapsed during a violent storm as the train passed over it, tragically killing all aboard. Engineer Benjamin Baker conducted one of the early wind engineering analysis on the design post-disaster and found that the design accounted for too low a wind load. In the 1940s, Danish engineer Martin Jensen began performing some of the first wind tunnel testing. with a boundary layer. He also modelled analytically the turbulence with the increasing wind speeds above the ground but found the coefficients obtained were different from those recorded in aeronautical wind tunnels. He discovered that the real wind had to be modelled accurately to get reliable values of the pressure and hence wind loading on the structures.
The progression to tall buildings took off in the mid-60s when the World Trade Center Towers were being designed. The engineers back then had less knowledge of the behaviour of tall buildings and performed wind tunnel testing at the National Physical Laboratory in the UK and in Colorado. Professor Jack Cermak of Colorado State University built one of the first large boundary layer wind tunnels designed specifically to investigate the effects of natural wind in the built and natural environments. Alan Davenport, the second father of wind engineering along with Jack, determined the important statistical variables that engineers needed to define and study. These brilliant minds gathered in Fort Collins, Colorado and worked out the basics of the science of wind engineering. These basics would then influence our modern building codes and standards, which were derived from the wind tunnel tests performed during this period.
These codes and standards state the pressure and the loads that are expected to be experienced by the structure and must be designed for in specifying the building envelope. This allows engineers and designers to estimate appropriate design loads in the absence of a building specific wind tunnel test. While generic to a degree, standards can also account for factors such as the surrounding terrain. The surrounding terrain is categorized by its level of exposure and this is accounted for in the estimated local boundary layer. E.g., in the United States, there are exposure categories B, for urban areas, C for open country, and D for open water. Simply put, these standards provide a quick and relatively simple way to estimate the loads and design standardized buildings.
A building is essentially an obstacle embedded in a flow field. This flow field is the air around us. Like any object in a flow field, its presence alters the flow around it, resulting in pressure differentials and hence pressure loads on the body. As the building gets taller, so too does the flow field change within the atmospheric boundary layer that the building resides in. The mercurial climate adds an element of complexity into the design, as the building should be able to withstand the worst that Mother Nature can throw at it while keeping their occupants safe and comfortable.
Tall
When assessing the aerodynamics of tall buildings, the purpose is to demonstrate sufficient reliability in the structural system and the building envelope. Will the building be safe, and will it perform as intended under the wind and pressure loads? The suitability of the structure to withstand the wind loads must be demonstrated, that it is not going to crack or fail under expected loads. Its serviceability also needs to be demonstrated. In civil engineering and structural engineering, serviceability refers to the conditions under which a building is still considered useful. The building should not drift or vibrate too much under loads as this has an impact on occupant comfort. The windows should not pop off or crack due to impact damage, prevalent in areas with strong wind environments such as Hong Kong with its typhoon season.
There are many facets of a building not often considered such as the elevator operation. Large pressure differentials within the elevator shaft and/or building sway of tall buildings can affect elevator functionality; its operating speed may have to be decreased, noise effects and issues with door operability (being unable to close or swinging open) can arise.
There are 2 methods of demonstrating this reliability: a standards-based approach that employs compliance to building standards, and the wind tunnel approach. Both are ways to quantify the expected loads experienced by the building and are often used in conjunction.
Short
While tall buildings may get the hype, the glamour and define a city's skyline, shorter buildings and their considerations are important too. Many relatively shorter buildings can serve as integral components of a company's supply chain, operations or provide essential services like hospitals, utilities, and communication lines.
Large shorter buildings can be immensely valuable, particularly mission critical buildings such as warehouses and data centres of businesses. The impact on its serviceability or worse, failure of these buildings can be devasting to the operations it supports. For example, on several occasions when tornados tore through the American Midwest, they took out many supply operation buildings of the major automotive manufacturers, severely disrupting operations, and product delivery. Similarly, for hospitals and data centres that require uninterrupted post-disaster occupancy and operation, the precise knowledge of the expected loads under extreme wind loads is instrumental in their safe and reliable design.
There has also been a large amount of effort in ensuring efficient cooling of data centres. Cooling, ventilation and removing of the heat is vital to the long-term operations of these buildings and these aerodynamic concerns fall under the Heating, Ventilation, and Air Conditioning (HVAC) systems.
Overall, a holistic view of the project must be considered. A large square floor is often more sellable and more valuable as compared to accommodating changes solely to optimize aerodynamics. This must be balanced against the cost of the modifications and against the opportunity cost on the structure. i.e., how much more usable floor space can be saved by not making these modifications. This process is a team exercise between the architect, structural and wind engineers. In standard buildings, the architect fixes most of the shape with the client and the structural engineers will perform a first check of the expected loads by referring to standards before a wind tunnel test is used to confirm that the design is adequate. Here is when the alterations are made to reduce the loads below the standards which can lead to savings. Big savings can be made particularly in the building envelope. For typical glass faced office towers, the building envelope can cost up to 30% of cost and savings here will lead to massive savings for stakeholders involved.
Aeroelasticity
The coupling of the aerodynamic forces and the structure's response can introduce aeroelastic phenomena. Commonly used in the context of aeroplanes and their lifting and control surfaces, buildings can experience such effects too. However, it depends on the structure in question.
When the overall wind loading is examined for the structural design, there is a choice between a rigid model or an elastic model. In a rigid model, the pressure distribution is measured together with the combined load at the base. The dynamic response of the structure is then introduced mathematically. This is done using a mechanical admittance function. The applied force is multiplied through the mechanical admittance function to obtain the responses of the building, in terms of expected loads on the structure. Aeroelasticity is a concern depending on whether the building is moving enough to alter the aerodynamic excitation mechanisms.
Most buildings which are short, stumpy, and not aerodynamic enough, are often positively aerodynamic damped. The movement of the building provides a damping effect. However, this is small compared to the inherent structural damping of the building and hence it is usually ignored. However, with tall and slender buildings, negative aerodynamic damping can occur, typically when vortex shedding occurs at the sides of the building. If the rate of the shedding coincides with the natural frequency of vibration of the building, a feedback loop can be set up such that the motion of the building further increases its level of excitation. It is usually not huge for most buildings except for rare cases such as an extremely slender building. Instead, aeroelastic effects are more commonly significant for smaller components of the buildings such as masts, sunshades and solar arrays as these objects are smaller, lighter, and hence more prone to unstable movement. It is more often the aeroelasticity of these components that are investigated as compared to the main structure.
Planning For Disaster
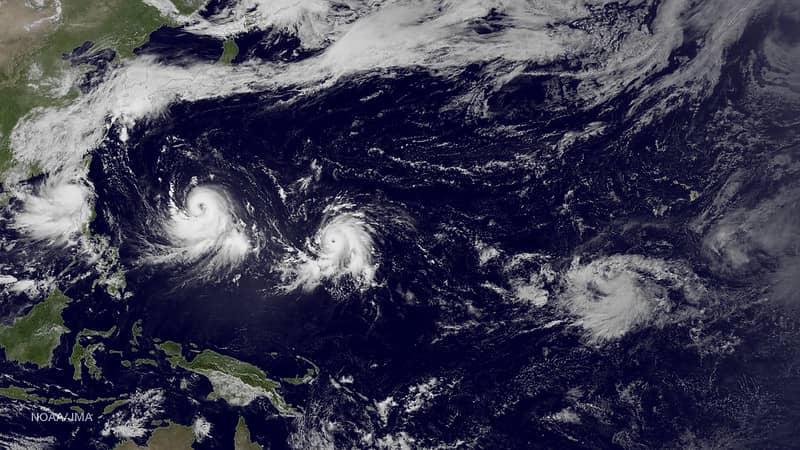
It is vital that our buildings can withstand the worst that Mother Nature can throw. While the wind and its direction may seem unpredictable, wind engineers have methodologies and tools to account for it. Site-specific wind climate analysis involves performing statistical fits by direction on years of meteorological data to obtain a statistical model of the local wind climate. In many places, the highest wind speeds will probably come from specific directions and designs must account for the probability of subsequent load effects. A statistical model of the wind climate is built and factored into the building design. Statistical meteorological studies of the local climate are invaluable as direction of wind is not considered in most standards. Without directions, one would have to consider the worst wind coming from any direction and may over-engineer the design. With statistical meteorological studies and wind tunnel testing, the statistically likely direction of the strongest wind can be designed and tested for.
Regions that are prone to typhoons (also referred to as tropical cyclones or hurricanes in different parts of the world) are typically situated in coastal locations as these meteorological events lose intensity as landfall is made. A typhoon Monte-Carlo simulation is performed to provide directionality. Using such statistical simulations, the directionality of typhoons/hurricanes can be relatively well predicted. With the direction from which they track known, using their rotational speed and predicting their translational speed from the storm's intensity, a decent directional model of the storm can be created! Unlike typhoons, tornados are a fundamentally different meteorological structure and there is an up-and-coming effort to define tornado loads in the US Wind Loading Standards next year. There is also interest in multi-hazard design standards which account for abnormal loads (flood surges, earthquake loads, typhoons etc.) in a more holistic manner, assuming these events and associated loads occur simultaneously instead of individually.
CPP provided wind engineering services for the building of the International Commerce Centre in Hong Kong and was instrumental to its design. "Its original alignment on a side of the building was unfavourable relative to the strongest typhoon wind direction. We convinced the project team to rotate the tower by about 20 degrees and that significantly reduced the wind loads."
Poor Aerodynamics
Poor designs can be split into two categories: an overall poor design or if it had been designed to outdated standards which are no longer sufficient for the current standards of building performance. When a wind engineer is called in to diagnose a problem, some forensic work must be done to identify the cause. They start with a known problem and then validate the issue through testing. From there, solutions can be explored. Common aerodynamic problems are excessive movement of the building and noise generation. The wind engineers will often work closely with the structural engineers to resolve such issues.
First, the original assumptions of the expected loads on the buildings are examined to ascertain their validity. As building standards are updated with time, older buildings may be designed to standards that are no longer acceptable. Building design relies on the predictions of dynamic properties such as the modulus of elasticity of the concrete, expected live loads and foundation flexibility. Naturally, these predictions are never accurate and when problems arise, they must be checked by taking real measurements. i.e., coring and testing a sample of the concrete to test for its strength and modulus of elasticity, examining the natural frequency of the vibration of the building etc. Next, wind tunnel tests of a model of the building and its current surroundings are performed. This step is critical especially if the surrounding architecture has changed since the time of construction as new developments could alter the flows in ways not accounted for in original design. Wind tunnel and CFD simulations can be used to verify that the proposed cause is correct.
For shorter buildings, if the cladding has detached, the wind event that caused that failure is examined by performing meteorological analysis of the actual wind speeds at the site. Many of these events happen at a micro-scale (thunderstorms with a few tornadic outbursts and local downburst in the middle). With a better understanding of the local conditions, the standards are examined to see if they are sufficient for them.
If a building is slated to be stripped down, repurposed, and redesigned, the process will follow a procedure like the one described above. The original design assumptions are re-evaluated to check for its relevance in accordance with current standards. Older buildings tend to be surrounded by more developments than they were when they were first built, which provides a lot of shielding from the wind. If a building is about to be re-cladded, the new design maybe tested in a wind tunnel to provide accurate data on the actual loads the new design should account for. Retrofitting of the building during this process is also possible although not a common occurrence.
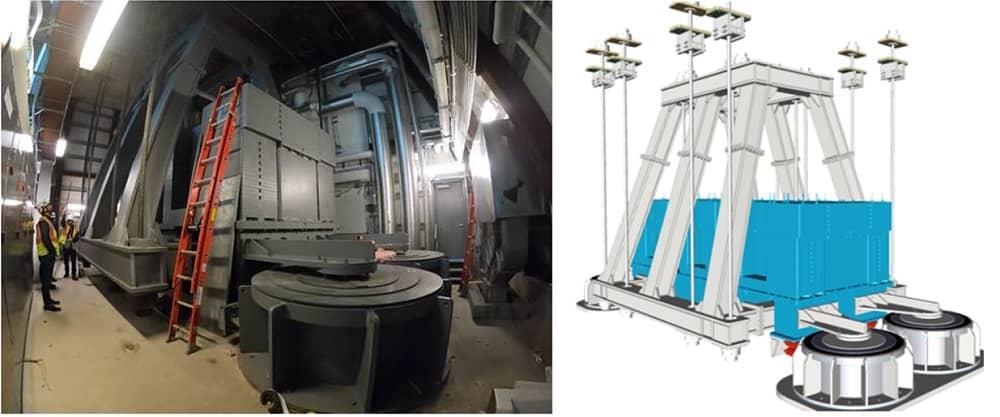
The proposed SRG Tower in Dubai is an example of a building that was re-designed due to its aerodynamics, standing at 460 meters high with a very small footprint. An initial wind tunnel test recorded large loads and responses. An estimated 2000 tonne tuned mass damper was proposed as the measure to control the excessive motion. This would have introduced massive cost and space requirements. Changes were made by the architect and structural engineer and CPP was hired to provide wind engineering services. "It was a great collaborative project with all stakeholders at the same table and with the aerodynamic considerations driving the architecture. Our proposals to improve the design received support from the architect and structural engineers. Several iterations of design were tested, and improvements made such that the initial proposed tuned damper was no longer needed! Little bits of aerodynamic modifications such as the inclusion of sky floors and sky gardens, corner shaping of the building and couple of others reduced the loads on the building."
Wind Comfort
The wind comfort criterion involves two factors: pedestrian safety and outdoor wind comfort.
From a safety perspective, people and objects like vehicles should not be getting blown over on the street. When studying pedestrian safety, there are criteria for wind speeds at which people typically get blown over. This safety criterion will state that critical speeds are only acceptable once or twice a year in abnormally strong winds (such as during a storm) and should not be expected on a regular day. From a comfort perspective, criteria for more commonly occurring wind speeds are available. If these wind speeds are exceeded for a threshold percentage of the time, the location is deemed unsuitable for certain outdoor activities. Wind comfort criteria differ for each activity such as but not limited to: standing, outdoor sitting, dining and walking.
With the COVID-19 pandemic encouraging the minimising of close contact indoors, there is greater emphasis on ensuring the wind comfort of outdoor spaces. People will likely avoid indoor spaces and mass transit and instead be outdoors and travel on foot or by bicycle. People want to sit and meet outside more. Restrictions on indoor sitting for restaurants have pushed people to dine outside. This pandemic has increased the demand for usable outdoor space as well as conversion projects. These spaces are usable only if they are comfortable, with the right level of wind passing through them. Developers can also adopt a more holistic perspective, accounting for wind and thermal comfort. What is deemed comfortable also depends on the location, as higher wind speeds in a hot and humid place like Singapore could be welcomed as compared to a place like Scotland. Wind comfort has become a revenue factor for buildings. Spaces rented to outdoor businesses such as restaurants must be usable and pleasant for customers. Getting this level of comfort right affects the attractiveness of the real estate and hence the return on investment for the building owners.
In relatively low-rise locations and if safety issues are not a main concern, wind comfort studies can be performed computationally only. If a safety issue is at hand, the wind tunnel will be used as well. The key objective is understanding the surroundings and the local meteorological environment and getting the statistical model right. "It is not as simple as simulating a handful of directions in a CFD model and identifying regions of higher wind speeds, that does not provide a full picture. You may have the highest wind speed coming from a rare wind direction, we must be able to state the percentage of time where it is acceptable for various wind speeds to occur. We typically have 16 wind directions to consider and integrate into the wind climate of the location to be able to confirm that the design of the site works for the intended use."
Taming The Wind
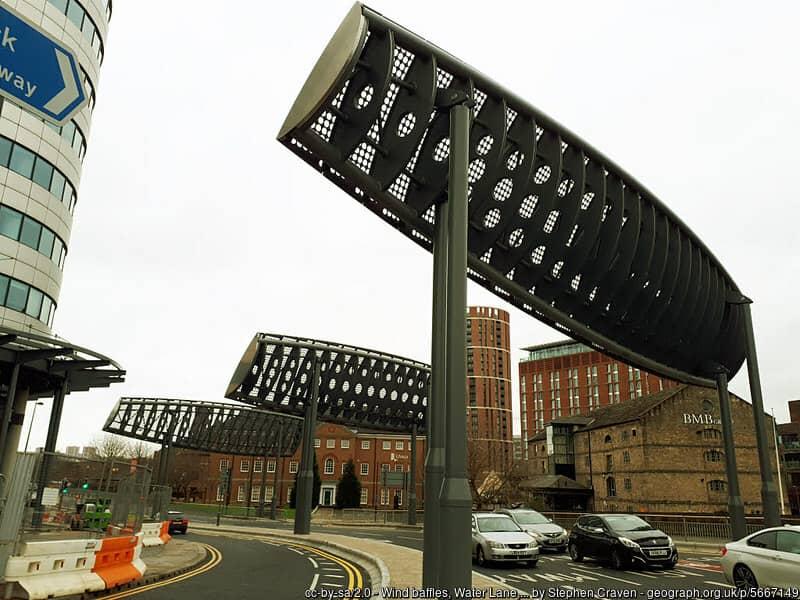
Wind engineers can employ a variety of methods to improve the wind comfort of the location. Some places can be improved with simple vegetation and the addition of baffles. Others may require a canopy, which can be difficult to install depending on the structural capacity of a building and what lies underneath it, as supports for the canopy may need to be driven into the ground. But to simply increase comfort, small wind baffle elements or temporary canopies can be added.
The Bridgewater Place skyscraper in Leeds is an example of a severely mismanaged wind comfort criteria. The presence of the Bridgewater Place skyscraper introduced dangerously gusting high winds at the base of the building. Numerous injuries were reported from pedestrians being blown over, including the tragic death of a doctor who was crushed by a truck that was blown over. Measures were eventually taken to reduce the strong winds. A large canopy around the plaza was added on the north end of the building. Big porous vertical wind screens were added around the end of the building. Across the roadway there are a series of baffles which spanned the roadway. Additions were needed outside the building site just to improve the wind conditions at the site. This is one of the more extreme examples of poor wind comfort. Tackling this issue has made extensive wind tests part of the proposed plans for future developments in the city. Given the wide footprint that modifications to accommodate wind comfort criteria can require, substantial cooperation is needed to resolve these issues. The inclusion of wind safety criteria in building standards by local authorities go a long way with regards to long term plans for urban development by providing consistency and coherency in the development strategies.
Wind comfort criteria have become a focus over the past few years and CPP handles several hundred projects per year. Different countries will have different levels of demand as these conditions are highly dependent on the meteorology of the location as a windy city will likely be more affected by these concerns. A coastal location in the North like New York along the east coast of the United States is much windier compared to Singapore. For a country like Singapore situated in the doldrums, its benign wind climate will likely have criteria that encourage breezes to boost pedestrian comfort.
Tools Of The Trade
Wind Tunnel
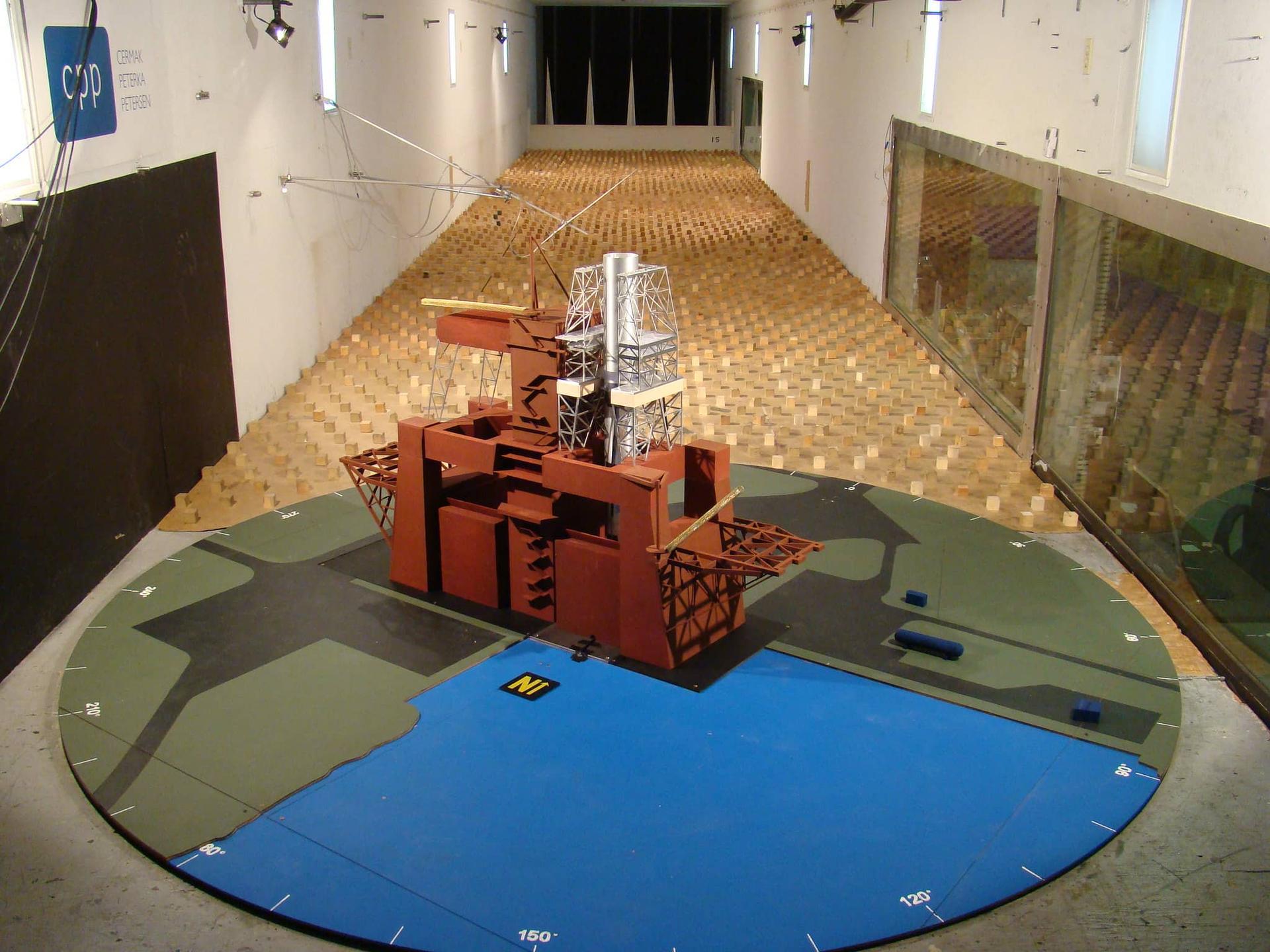
Wind tunnels, more specifically atmospheric boundary layer wind tunnels are a staple tool in wind engineering. They differ from the usual aeronautical wind tunnels used in automotive and aerospace applications as they are specially designed to recreate the atmospheric boundary flow seen by the building in its locality.
The use of a wind tunnel is dependent on the project. In certain locations where a new building is proposed, local planning standards will dictate that a wind tunnel test is needed to demonstrate no detrimental effects introduced by the new building on the pedestrian wind environment. For projects situated in these locations, wind engineers will be involved from the early concept planning to ensure the proposed building is not detrimental to the wind environment before the rest of the design and construction take place. For extremely tall and slender buildings, an early wind tunnel test at the start of the project helps stakeholders get a feel of the loads and responses expected for the first design of the building from which further shaping in close collaboration with the design team can take place to optimize its aerodynamics.
Dr Denoon, who worked on the wind analysis of the Marina Bay Sands Hotel, describes the process: "For a building like the Marina Bay Sands, a more detailed analysis of the upwind terrain was needed and performed using a wind tunnel test. The roughness of the surrounding region is categorized over different lengths within a 15-20km radius from the site. These categories then allow us to calculate and characterize the boundary layer expected at the site. i.e., the profile of wind speeds above the ground, the turbulence intensity, and the gustiness of the wind. The extremely local surrounding was considered using a detailed model in a turntable atmospheric boundary layer wind tunnel. All the buildings expected to significantly affect the flow are modelled accurately within a 400-600 m radius of the site."
The use of wind tunnels in the design process requires their results to be validated, to demonstrate they are indicative of real flow effects. This can be difficult to perform with sufficient data, however efforts have been made by institutions such as the Building Research Establishment in the UK. In the 1970s, they constructed a full-scale house installed with pressure sensors and collected a few years of data on it. The Agricultural Institute at Silsoe built a full-scale barn around the same time and tested it similarly. These datasets were then used to validate the wind tunnel predictions. This is an ongoing process in the wind engineering community.
Computational Wind Engineering (CWE)
CWE is the application of CFD within a wind engineering environment, where meteorological statistics are integrated into the analysis as well as knowledge of the atmospheric boundary layer flows. CPP utilises a range of commercial CFD software, the open-source OpenFOAM package, as well as proprietary in-house packages that run over these applications specifically designed for wind engineering. For most wind engineering applications, unsteady simulations are ran. A steady RANS model is great for predicting mean flow fields and providing a quick overview of the mean loads. However, as most effects of interest are unsteady in nature, the turbulence structures must be simulated accurately for the output to be useful.
While there exist standards and guidelines that govern wind tunnel usage to ensure its output is reliable for wind engineering applications, in the computational domain, no such standard yet exists for simulations involving the atmospheric boundary layer. Some standards still refer to CWE as an unacceptable route to design and compliance while others may state that CWE can be used only if a validation by wind tunnel testing is performed.
"This is a current area of development within wind engineering community. We are writing something that will guide future use of CWE, determining what measures must be taken for the analysis to be used reliably. The pathway is being built for future acceptance of computational methods."
Which Is Better?
The purpose of testing, be it in a wind tunnel or computationally, is to obtain accurate turbulent structures and hence accurate wind loads. The most common way to model it in a CWE analysis is to model an actual wind tunnel to generate the required boundary layer. This computational setup is costly by itself. Yet to be included is the high-resolution cost needed for the surrounding buildings to get the precise interaction of the flow structures with the building correctly! The wind tunnel is nothing but a big analog computer. For a typical test, 36 wind directions are tested, with each taking about 2 minutes to run. To examine similarly with any degree of resolution computationally will take a day or two for each wind direction. This also does not include the verification of the computational results. As such, the wind tunnel is still the better way to design a building.
CWE techniques alone can be used for pedestrian wind studies but only for those related to wind comfort. When it is related to safety, which involves understanding the gustiness of the wind, the wind tunnel is the only reliable way to examine these effects. "To be able to simulate reliably the loads on buildings for safety applications with CWE, the industry still has quite a few years to go before it is a reliable and economical approach”.
Wind Engineering Moving Forward
Building standards and codes will be constantly iterated upon as engineers continue to design, test, and evaluate new buildings. While the wind tunnel is a very mature technology, CWE will continue to improve faster as modern results get validated. With stronger reliability in CWE techniques, its quick turnaround time will make it an invaluable tool to guide early design directions. The application of the datasets collected will also improve as the industry explores performance-based wind design. This combines traditional wind tunnel techniques and structural design and melds them together to create more efficient designs never thought possible.
"We have published a pre-standard recently that defines the methodologies of performance-based wind design. For example, some aspects of a building’s structure will be allowed to go beyond the plastic range only if we can show it retains overall structural reliability and we can show there are enough alternative load paths."
Interesting links:
CPP Wind Engineering and Air Quality Consultants
Run Your Own Simulation