Aerodynamics is one of the most important tactics for improving performance, which in most cases is to go faster. So what is the relationship between aerodynamics and speed, and how do different industries optimise aerodynamics to increase speed? This article provides a detailed explanation of the theory behind these questions as well as an insight into how the aerodynamics of runners, cyclists, motorcycles, cars and aircraft are all improved.
Aerodynamic drag and speed
The Bugatti Veyron is one of the fastest cars ever made. The original 987bhp (1,001ps) version clocked a top speed of 407kph (253mph) in 2006 [1]. While the revised 2010 Super Sport increased power output to 1,183bhp (1,200ps), boosting the top speed to 434kph (270mph) [2]. So in this case, it required nearly 200bhp to gain 17mph.
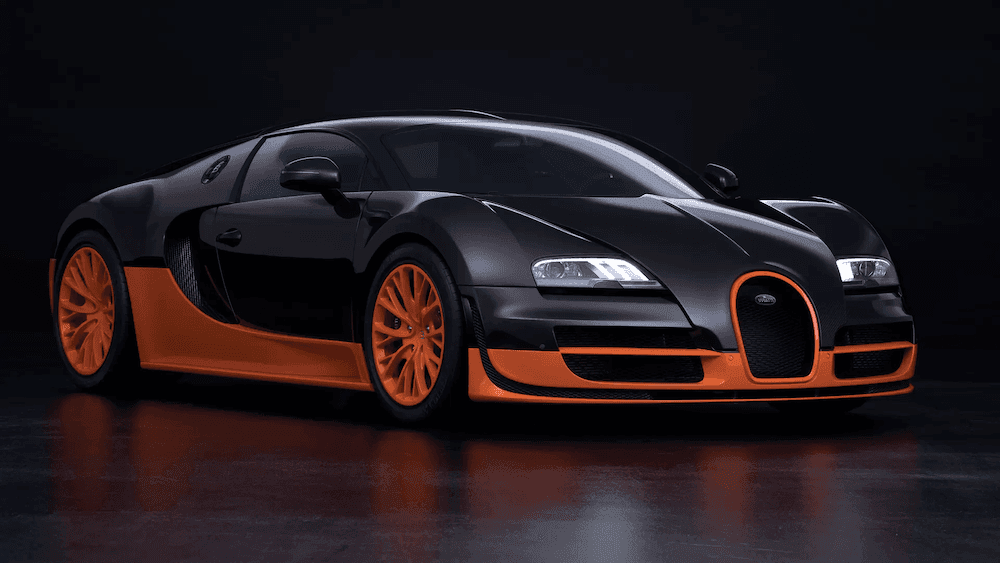
The similarity of the underlying cars demonstrates nicely the problem of aerodynamic drag in the hunt for speed. The standard force and power equations show that drag has a squared relationship with speed, whilst power has a cubed relationship.
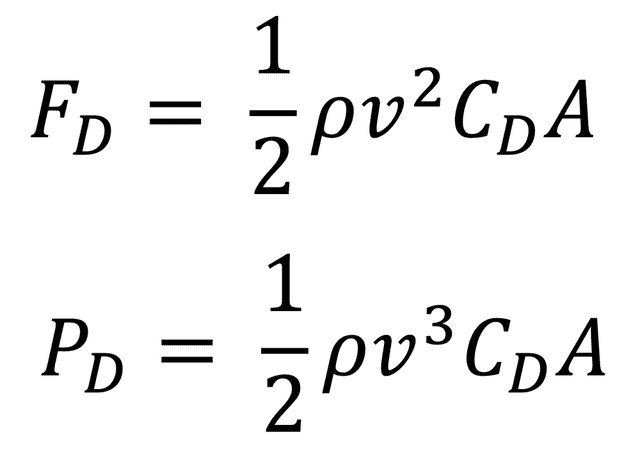
Where: Fd = drag force (N), Pd = power (W), ρ = density (kg/m³), v = velocity (m/s), Cd = drag coefficient, A = frontal area (m²).
Whether power comes from internal combustion, electricity or a person, it is difficult to find the increases needed to make small gains in speed. Instead, engineers look to the other three variables in these equations: air density, drag coefficient and frontal area.
Engineering drag reduction
Drag coefficient and frontal area are physical aspects of design that are well understood. Wind tunnels and CFD allow engineers to work with athletes and design vehicles to produce forms that reduce the force required to overcome aerodynamic drag.
Air density is a less obvious variable that can be used to enhance designs and optimise performance. Cyclists breaking the world hour record will choose temperature controlled velodromes at altitude to reduce the density of the air they are pushing through. Lower air density also means less oxygen which reduces the available power, so there is an important compromise to be found.
How aerodynamics affects the speed of runners
Human athletes are a good starting point to investigate how aerodynamics are used to increase speed. Analysis suggests that around 90% of the energy that Usain Bolt expended during his 9.58s record 100m run was required to overcome drag [3].
As a result, sportswear has been developed to be lighter and minimise drag. The original Nike Swift Suit worn by Cathy Freeman in 2000 has now evolved into arm and leg sleeves with 3D moulded blades that aim to reduce skin friction and wake size.
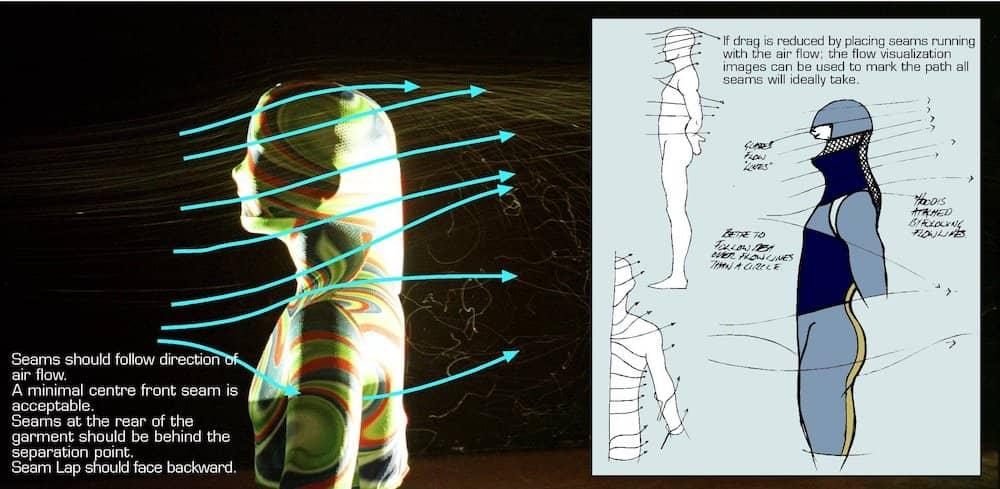
A moving human being is a difficult subject to model. Individual shape, size and running style produce different air flows and wakes. Elite athletes will have bespoke mannequins for wind tunnel testing to precisely locate drag reducing features.
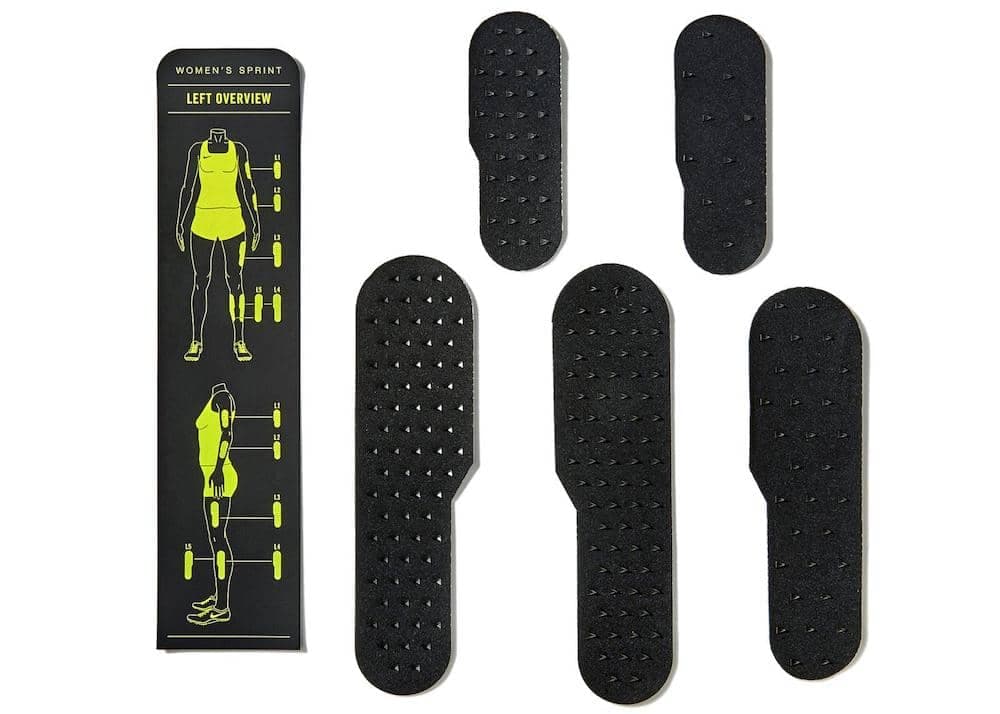
International competitions have strict clothing regulations to limit the impact of technology. Despite this, teams will go to impressive lengths to push the boundaries of performance. This was proven by the INEOS 159 Challenge where every available technology was exploited to help Eliud Kipchoge run the first sub-two-hour marathon. The team optimised the formation of the pace runners using wind tunnels and CFD, while an electric pacecar calculated the precise speed (to within 0.1kph, 0.06mph) required to guide the runners to the sub-two hour target.
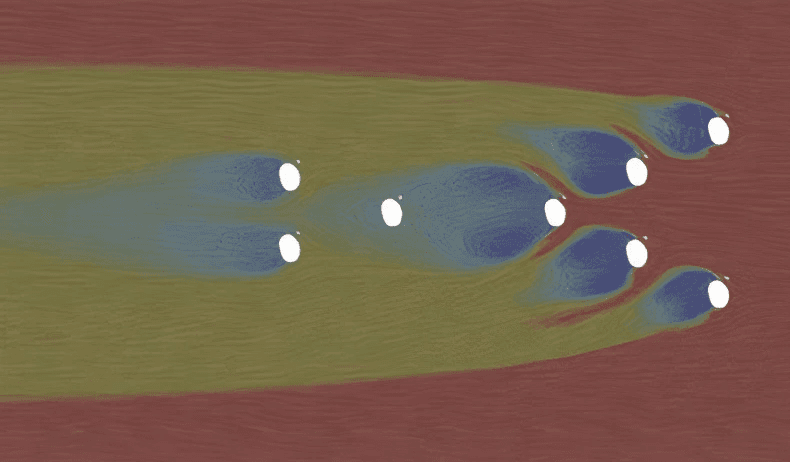
How aerodynamics affects the speed of cyclists
Cycling has perhaps benefitted the most from recent aerodynamic advances. In part this is due to the capabilities of the latest Aero bikes and aerodynamic clothing, but also because it lends itself well to wind tunnel and CFD analysis. The relatively fixed rider postures can be easily modelled, analysed and optimised to reduce drag.
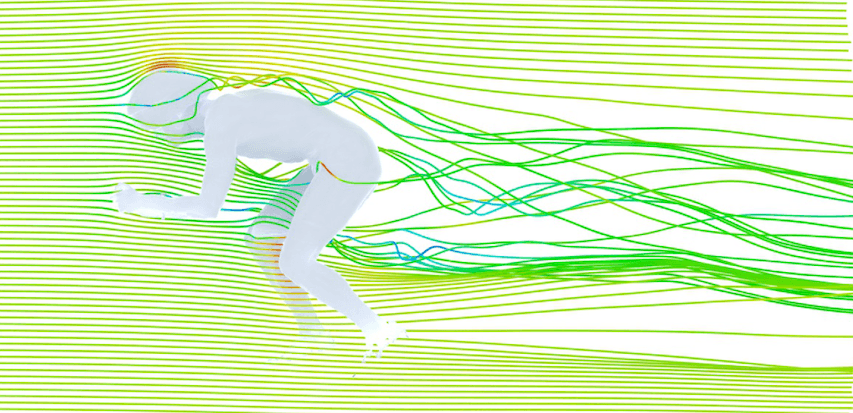
As with most innovations in sport, regulation changes are not far behind. Textured arm and leg sleeves can be used to reduce surface drag and control leg shape. In cycling, short lengths are controlled and oversocks banned, so teams wear long socks instead. While regulations continue to limit sock length, teams often turn to new innovations, which we saw at the Tokyo 2020 Olympics where the Danish cycling pursuit team applied kinesiology tape to their shins.
The importance of drag to cycling performance is perhaps best demonstrated by the typical speeds achieved in different disciplines. A Tour de France rider cruises at 48kph (30mph) sharing drag in a peloton. The world hour record average speed by a single rider in a velodrome is 56kph (35mph). The drag-assisted world record on a custom bicycle is 295kph (184mph) [4].
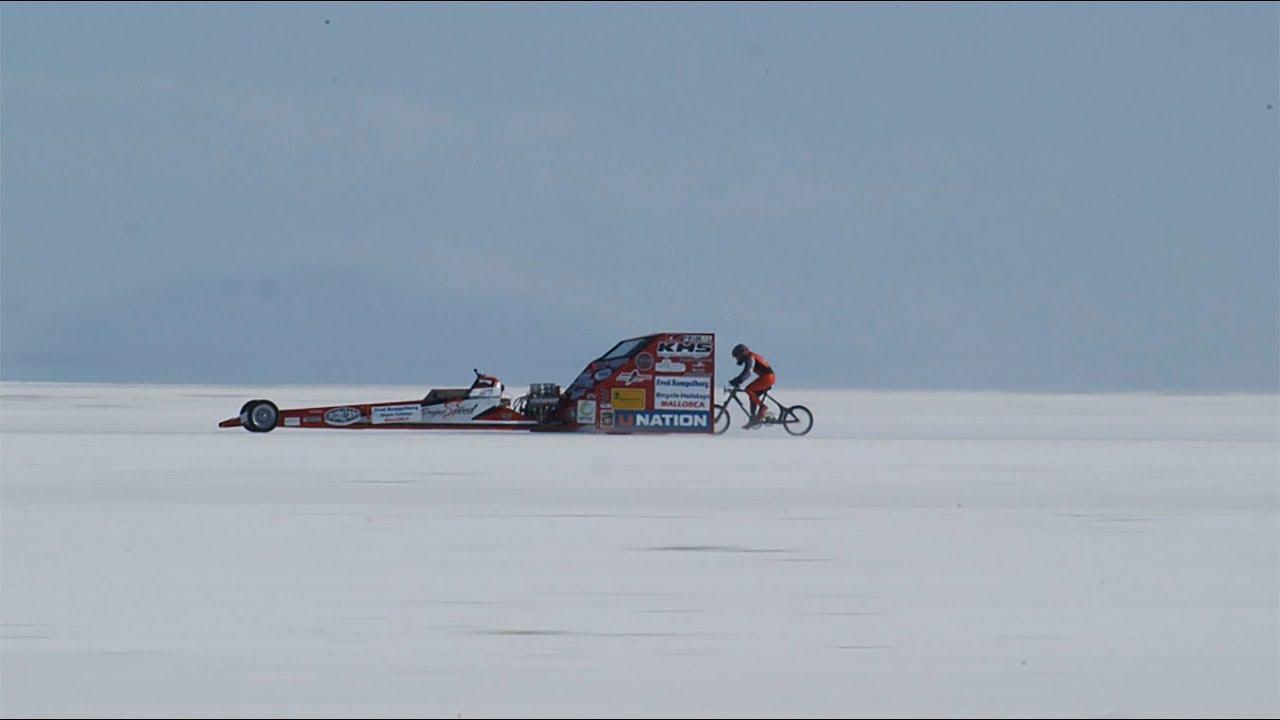
Denise Mueller-Korenek sets the bike land speed world record
How aerodynamics affects the speed of motorcycles
The exposed nature of a motorcycle leads to turbulent flows, reverse flows and separation which increases the total drag force the bike has to overcome. So, despite motorcycles having a frontal area approximately two thirds smaller than an average car, its drag coefficient can range from 0.5 to 1.0 which is almost double that of a modern car [5].
Similar to cycling, rider position and bike design are key to minimising drag. However, a recent trend has been to increase downforce by adding fairings and winglets to the front of motorcycles to increase the load on the front axle and optimise load transfer. This was one of the tactics employed by Ducati in MotoGP over recent years.
In terms of aerodynamic innovation, one of the most interesting motorcycle designs is the Voxan Wattman. This electric motorcycle set the world record in 2021, achieving a speed of 283mph (455kph) with Max Biaggi [6]. The removal of the requirement to corner results in a very different bike design and low drag riding position.
For example, three sets of bodywork were used to ensure maximum performance regardless of the wind conditions. This bodywork included a streamlined full fairing, a fairing with an exposed tail and a naked version. Furthermore, the seat was raised by 65mm which helped flatten Biaggi’s back and reduce drag.
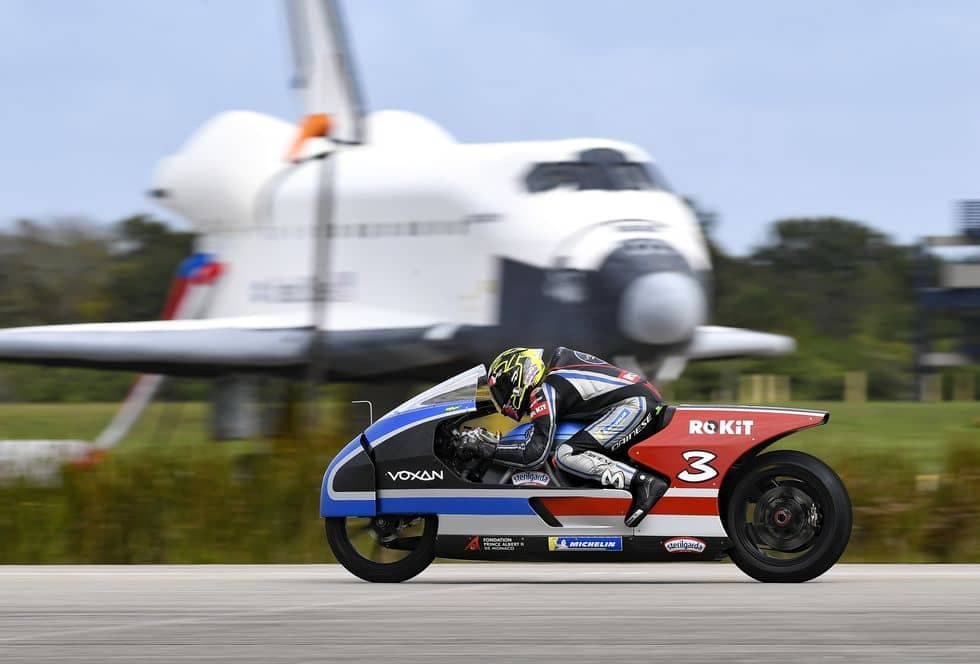
How aerodynamics affects the speed of cars
Top speed has long been a priority for road and racing cars. Before wind tunnels and CFD, streamliner bodywork was handcrafted to minimise drag. The 1930s Mercedes versus Auto Union battles saw innovative bodywork fitted to usually open-wheel cars.
Modern supercars overcome the problem of low drag and high speed instability with careful aerodynamic design in wind tunnels and CFD. The Lamborghini Rayo project used AirShaper to optimise the drag and downforce of the front splitter, underfloor and rear diffuser. Air curtains were also added to shield the front wheels to minimise drag. This optimised bespoke bodywork delivered a 300mph (482kph) version of the Lamborghini Huracan.
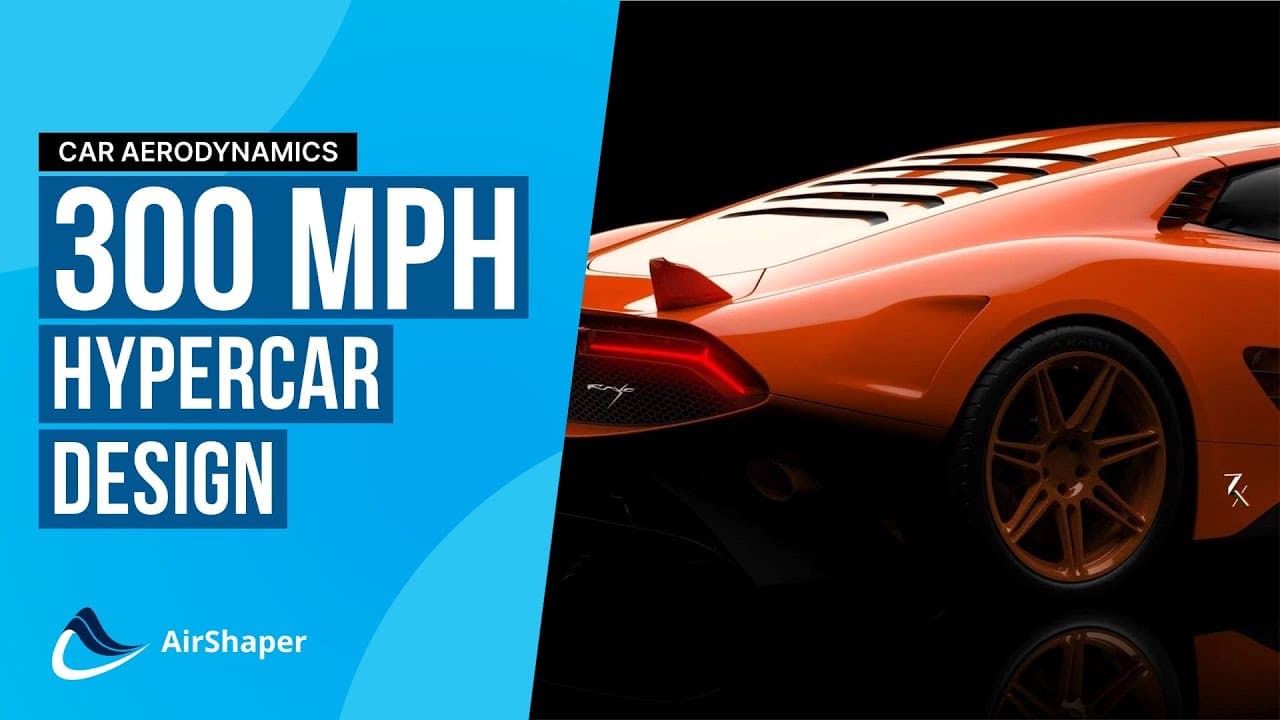
The Bugatti Veyron was a game changer for top speed and introduced the concept of active aerodynamics to road cars. Moveable wings and variable cooling exits allow cars to perform well in all conditions, generating high downforce during cornering whilst still achieving fast straight-line speeds.
The technology seen on these ground-breaking hypercars often filters down to more conventional applications. A common feature for high-speed configurations is to deploy a small spoiler. This is a drag reduction device that generates downforce to retain stability. In fact, Audi had to add a spoiler to its Audi TT to solve some high speed stability issues.
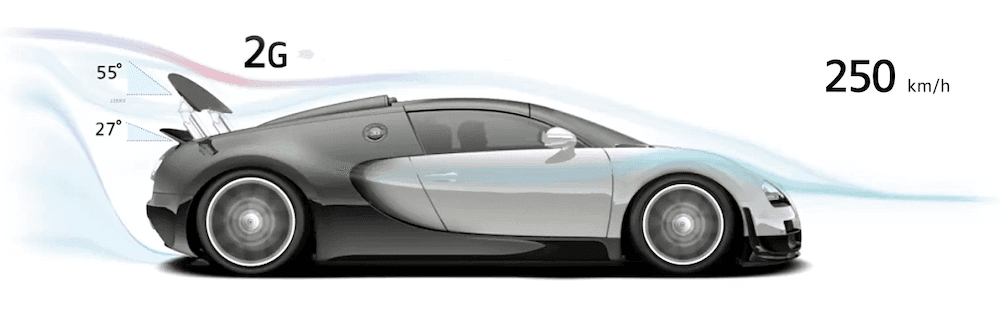
The Veyron was originally considered impossible to improve because it was such a difficult engineering exercise. Bugatti however, once again took on the challenge and developed the Chiron Super Sport which, at the time of writing, was the world’s fastest car, clocking 304mph (489kph) [7].
Although, it is the drive for efficiency of electric vehicles that is likely to offer the next batch of aerodynamic innovations. The Lotus Evija for example, takes advantage of packaging opportunities that an electric powertrain has to offer. A flat battery pack and in-wheel motors eliminate the engine, transmission, cooling system and fuel tank that normally make up much of the frontal area of a car. This offers more opportunity to exploit the underbody and through-body flows.
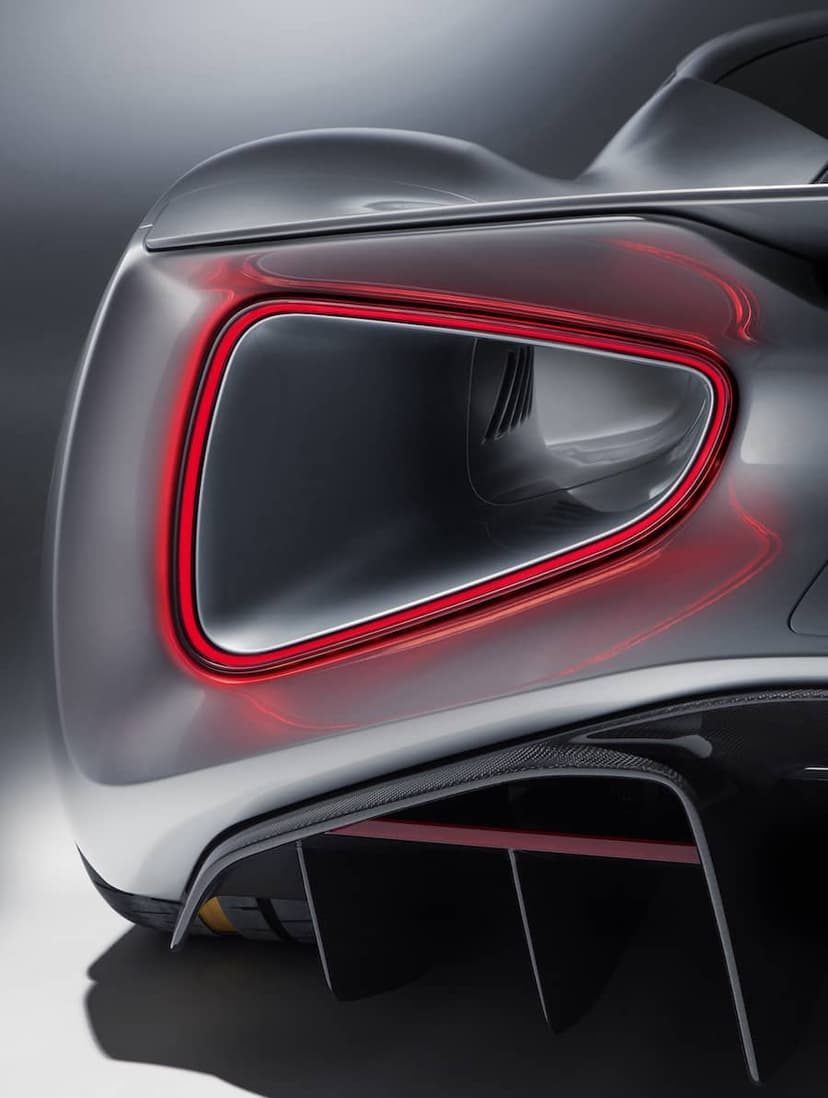
How aerodynamics affects the speed of aircraft
The latest hypercars represent the height of development of high-performance ground vehicles, with the fastest now running into compressibility effects, which are well known to aerospace engineers. Aircraft design accounts for air density in multiple ways. Altitude is obviously a key variable, but speed itself starts to have a significant effect. This is what makes machines like the North American X-15, the Lockheed SR-71 Blackbird and Concorde such engineering achievements.
At lower speeds, air is usually considered to be incompressible. As a result, air density is defined by altitude, temperature and humidity. As aircraft approach speeds beyond Mach 0.3, and particularly Mach 1 which is generally referred to as the speed of sound, compressibility becomes an issue.
In this transonic region, air molecules can no longer move quickly enough to move aside for the wings and fuselage. Instead, the air is locally compressed and becomes more dense. The rate of change of density rises rapidly above Mach 1, so much so that it increases with the square of the speed which in turn increases lift, but also drag. This higher drag means even more power is required to go faster. Together with the aerodynamic consequences of the shock waves produced at high speeds, this effect is called the sound barrier.
The sonic boom has been largely responsible for making supersonic airliners unworkable over land, diminishing the business case for a Concorde replacement. High-supersonic aircraft like the SR-71 and proposed hypersonic aircraft have problems with aerodynamic heating and engine performance that make them very difficult to design and operate.
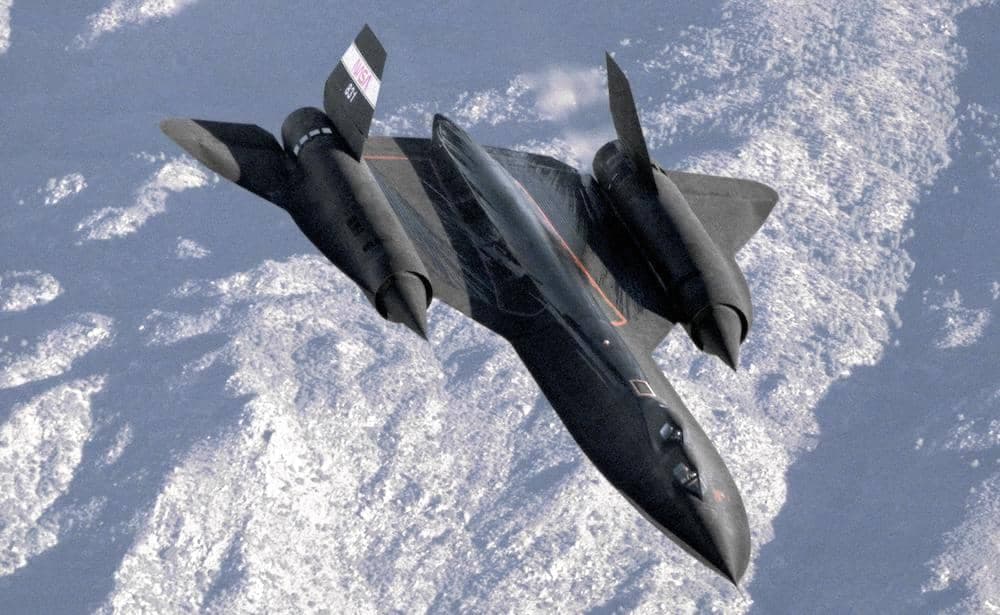
A common feature of supersonic aircraft of the 1970s is the swing wing, from the F1-11 to the Panavia Tornado. Like the active aerodynamic wing configurations on a Veyron, these are used to overcome the compromise between low landing speeds and high cruising speeds. At low speed, a straight wing is preferred to generate lift and minimise take-off and landing distances. At high speed, these generate too much lift and drag. Sweeping them back reduces drag for speed and fuel efficiency purposes.
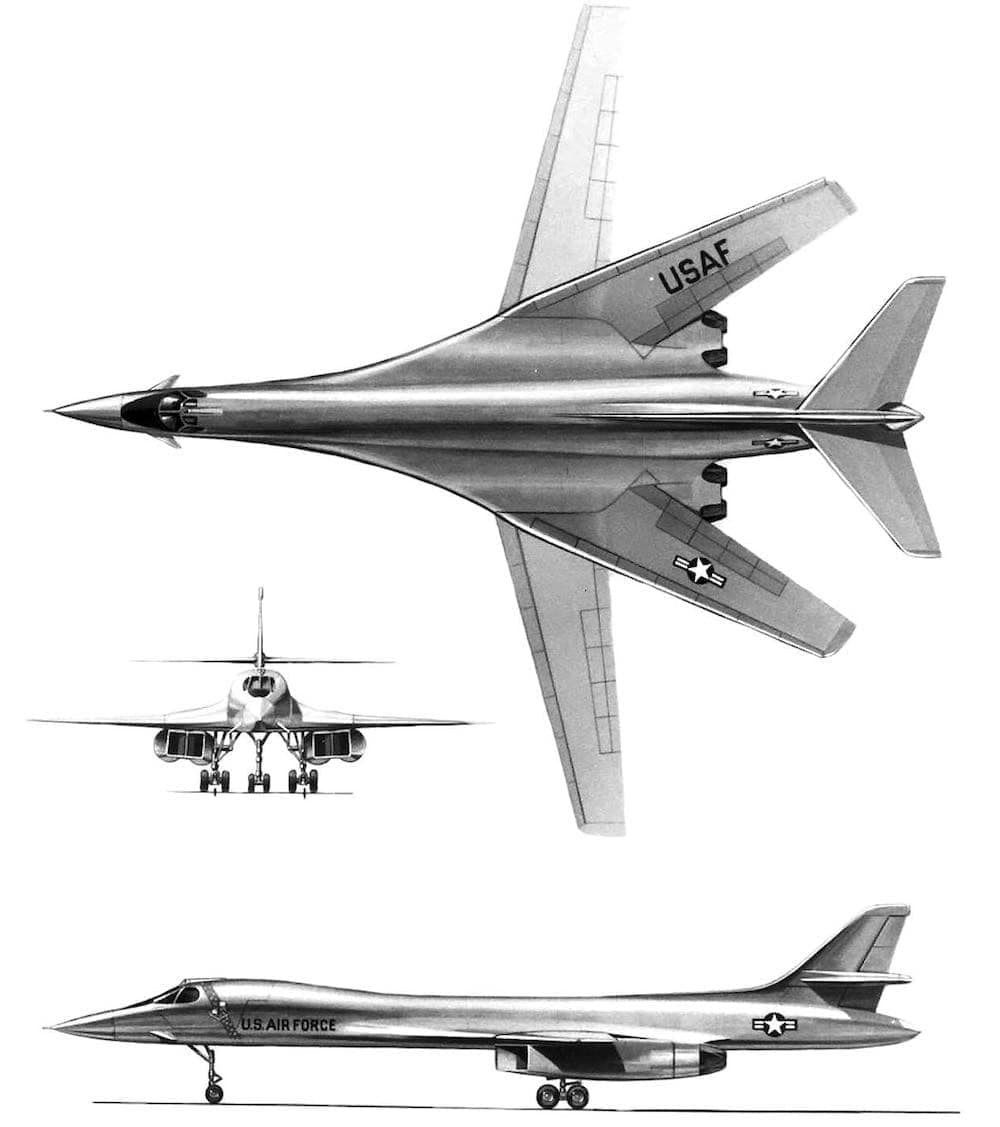
These types of aircraft were largely a product of the Cold War where innovation in drag reduction was required to outrun other aircraft. In 2022, drag reduction is still very much a priority to maximise speeds whilst maintaining maximum fuel efficiency.
Similar to vehicles, the next developemt step for aircraft is switching to electric as well as manned hypersonic vehicles. To understand the future of aviation, AirShaper's CEO, Wouter Remmerie, interviewed NASA to discuss new aerodynamic concepts and their X-57 experimental X-plane. It will be interesting to see the aerodynamic solutions to the future challenges in aircraft design and propulsion, but one thing is for sure, CFD simulations and wind tunnel testing will play an important role in solving them.
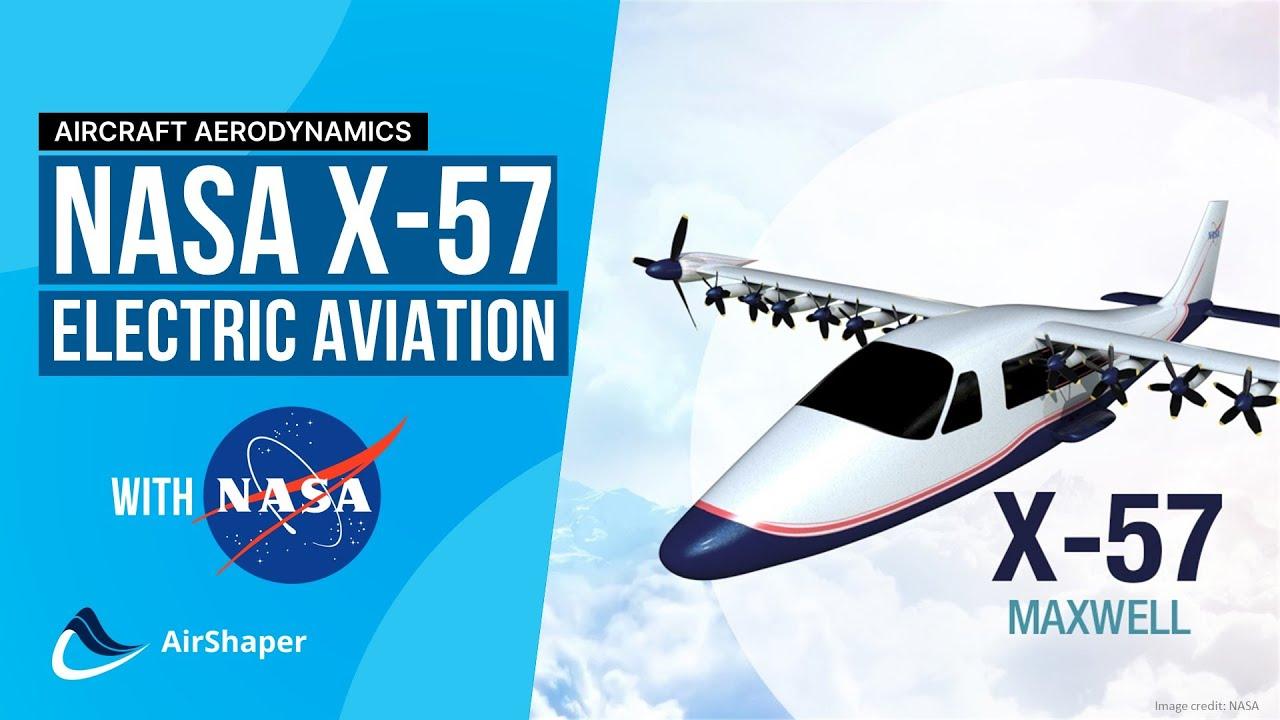
Interesting links:
- Detailed analysis of world hour records
- Wind tunnel testing of speed skiing posture
- Effects of altitude on Formula 1 car aerodynamics
- AirShaper analysis of the Lotus Evija
- A history of SR-71 Blackbird development
References
[1] 2006. Technical Specifications Bugatti Veyron 16.4 [Online]. Bugatti.
[2] 2006. Technical Specifications Bugatti Veyron 16.4 Super Sport [Online]. Bugatti.
[3] 2013. Physics of running fast: Scientists model 'extraordinary' performance of Bolt [Online]. Institute of Physics.
[4] 2019. Fastest cyclist ever: Setting a World Record speed of 183mph on a bicycle [Online]. Cyclist.
[5] 2020. How to improve motorcycle aerodynamics [Online]. AirShaper.
[6] 2021. Voxan and World SBK Champ Max Biaggi smash Electric Motorcycle Speed Record [Online]. BikeSocial.
[7] Bugatti Chrion Super Sport 300+ [Online]. Bugatti.